INTRODUCTION
Heart insufficiency is one of the main causes of mortality in patients with ischemic myocardiopathy. Recently proposed therapeutic options, both clinical and surgical, have as their main objective to treat only the consequences of the myocardial infarction and not the basic cause, which is the loss of the contractile cell, the cardiomyocyte.
Despite some authors suggesting that there is mitotic division in the heart [1], the great majority of the cardiomyocytes do not have a regeneration capacity after myocardial infarction and so, when this occurs, there is deterioration in the contractile activity and when the area affected is great, ventricular remodeling can occur. Recently, recovery of the contractile function has been evidenced using cell therapy, making this a viable therapeutic option. However, the choice of which cell type is best is still under discussion. The two cell types that are most being utilized are skeletal myoblasts and bone marrow cells.
Skeletal myoblastic cells have proved to be feasible in several experimental studies [2-6] and one clinical study [7], as they were differentiated in the skeletal fiber of myocardial fibrosis. However, there is still controversy in respect to the lack of differentiation of these cells in cardiomyocytes, an absence of a connection between the transplanted cells and the native cardiomyocytes and their arrhythmogenic potential. A multicentric study called MAGIC is being undertaken which may clear up some pending doubts.
On the other hand, bone marrow cells are pluripotent and have a capacity of differentiating themselves from the dependent medium [8]. Bone marrow cells can undergo two processes of differentiation [9]: mononuclear cells (non-differentiated) and multinuclear cells. One of the mononuclear cells is the hematopoietic cell, the cell that produces the blood cells (lymphocytes, eosinophils, basophils, neutrophils, red blood cells and platelet cells) and the mesenchymal, which may produce the muscle cells, hepatocytes, osteocytic cells, adipose tissue, chondrocyte and stroma.
Some studies report an improvement in the heart function after bone marrow stem cell transplantation to the myocardium [10], others, a differentiation in the cardiomyocytes [11] and others only an angiogenic potential [12].
Some studies utilized mesenchymal cells [13], others, hematopoietic cells [14] and others mononuclear cells [15] with different functional and anatomopathological results. There are methods and distinct costs involved in the isolation of these bone marrow cell fractions. Thus, the objective of this work was to compare the functional and anatomopathological results of mononuclear and mesenchymal stem cell transplantation in the infarcted myocardium with ventricular dysfunction.
METHOD
Experimental profile - Myocardial infarction
All experiments were performed in the cell therapy laboratory of PUCPR according to the "Guidelines on the Care of Animals" the guiding principles approved by the American Society of Physiology.
Wistar rats with weights of from 250 to 300 grams were intraperitoneally anesthetized using 50 mg/kg ketamine and 10 mg/kg xylazine. The animals were submitted to endotracheal intubation, without exposure of the trachea and to mechanical ventilation at a frequency of 60 cycles/min and a volume of 2.5 mL ("683" Harvard ® Apparatus, Inc., USA).
All animals were submitted to left lateral thoracotomy and ligature of the left coronary artery with 7.0 polypropylene thread (Ethicon®, Inc., Somerville, NJ) was achieved inducing infarction of the left ventricle anterolateral wall. The effectiveness of the procedure was proved when there was a change in the color of the left ventricular wall. The mortality rate of the animals submitted to this procedure was 40%.
Seven days after myocardium infarction, the animals were again submitted to anesthesia using 50 mg/kg ketamine and 10 mg/kg xylazine intraperitoneally and a bidimensional transthoracic echocardiographic evaluation was made [Hewlett Packard Sonos model 5500, with S12sectorial (5-12 mHz) and 15L6 limiar (7-15mHz) transducers], allowing an analysis of up to 160 Hz, developed for the ultrasonographic study of small animals. The transducer was placed in the left anterolateral portion of the thorax and the heart was imaged as a bidimensional axial view of the left ventricle with the mitral and aortic valves and the apex in the same image. The digital conversion of the image was obtained by a delimitation of the interventricular septum and the left ventricle posterior wall. Subsequently, the following measurements were taken: final systolic and diastolic surfaces, final diastolic and systolic lengths of the left ventricle and heart rate to calculate the final systolic volumes (LVFSV, mL) and diastolic volumes (LVFDV, mL) and the left ventricle ejection fraction (LVEF %). All the dimensions were blindly measured three times by the same echocardiologist, after which the mean of each parameter was calculated. Only animals with left ventricle ejection fractions of less than 30% were included in this study. The animals that presented ejection fractions greater than 30%, corresponding to 27% of the total animals, were excluded of the study.
Then the animals were divided into three groups: 1) Control Group (CG) - consisting of 21 animals, 2) Mononuclear Group (MO) - consisting of eight animals and 3) Mesenchymal Group (ME) - consisting of 13 animals.
Preparation and isolation of the cells
Bone marrow Aspiration
The animals were anesthetized, placed in the lateral decubitus position and subsequently antisepsis was performed.
Bone marrow was aspirated through multiple punctures of both posterior iliac crests using 5-mL heparinized syringes (liquemine 5000U/mL). The material was sent to the Cell Culture Experimental Laboratory to isolate the mononuclear cells. For each 100 mL of culture medium (DMEM) l mL of heparin was utilized.
Isolation of the mononuclear cells
The bone marrow suspension was diluted in essential Dulbecco's modified Eagle medium (DMEM) and slowly isolated by the Ficoll-Hypaque density gradient method (density = 1.077 g/mL), according to Boyum 1968. The material was centrifuged at 1400 rpm during 40 minutes. The ring of mononuclear cells, located in the interphase, was removed and passed to a conical tube, containing 20 mL of DMEM. The cells were washed two times at 1500 rpm for 10 minutes and resuspended in DMEM. A cell count was performed in a Neubauer chamber and the cell viability was verified utilizing Tripan blue stain.
Culture and expansion of the mesenchymal cells
The cells were placed on plaques at a concentration of 500,000 cells/mL in 25-cm2 cell culture flasks in 5 mL of DMEM supplemented with 10% fetal bovine serum, 1% penicillin and streptomycin sulphate and 10 µL/mL of insulin-like growth factor (IGF-I, Sigma - USA)
The bottles were placed in an incubator with 5% CO2 tension at 37 ºC and the medium was changed when there was cell confluence. The adherent cells were dissociated utilizing 0.25% tripsin-EDTA (Gibco-USA) and again placed on plaques for cell expansion for 14 days (Figure 1).
Transplantation of the cells
Eight days after myocardium infarction, the rats underwent cell transplantation. The animals were anesthetized with 50 mg/kg ketamine and 10 mg/kg xylazine and were submitted to median transsternal sternotomy and the adherences were dissected. The area of myocardial fibrosis in the left ventricle anterolateral wall was identified. An injection of the bone marrow cells was made in the left ventricle anterior wall, as follows: 1) Control Group (n= 21), received 0.15 mL of culture medium in the infarction region, 2) the Mononuclear Group (MO) (n=8) received 3 x 106 of mononuclear cells and 3) the Mesenchymal Group (ME) received 3 x 106 of mesenchymal cells (n=13). All the animals received 15 mg/kg/weight/day cyclosporin soon after the injection of the cells as the transplantation was heterologous.
Functional analysis
One month after the transplantation, a functional analysis of the left ventricle was performed using the same parameters measured by echocardiography as previously described. With the animals anesthetized, the transducer was placed in the left anterolateral portion of the thorax and the heart was imaged from a bidimensional axial view of the left ventricle, with the mitral and aortic valves and the apex in the same image. Digital conversion of the image was obtained delimiting the interventricular septum and the left ventricle posterior wall. The left ventricle final diastolic volume (LVFDV, mL), the left ventricle final systolic volume (LVFSV) and the ejection fraction (LVEF, %) were calculated using classical formulae. All echocardiographic analyses were blindly performed three times by the same echocardiologist and a mean was calculated. After the procedure, the animals were sacrificed using an overdose of anesthesia.
Histological analysis
The hearts were removed and washed in PBS (Gibcco-USA) and cryopreserved in liquid nitrogen. Eight-ìm cross-sectional slices were acquired by cryostat (Leica, 1850 model). The samples were morphologically analysed using the Gomori Trichrome method.
Statistical analysis
The results were expressed as means ± standard deviations and p-values < 0.05 indicated statistical significance. For intra-group comparisons between the initial evaluation and the evaluation after one month, the Student t test for parallel samples was used. After evaluating the homogeneity of the variances and the normal distribution of the data, a comparison among groups in the initial evaluation was achieved using variance analysis. For the comparison of the one-month results among the groups, covariance analysis was used with the initial results considered as co-variables. The least significant difference (LSD) test was used for multiple comparisons. The non-parametric Kruskal-Wallis test was used for the comparison of groups, when appropriated.
RESULTS
Echocardiographic analysis
Analysis of the groups separately
The left ventricle ejection fraction (26.84 ± 7.05% versus 21.79 ± 8.77% versus 26.62 ± 7.34%; p-value = 0.2505), the left ventricle final systolic volume (0.46 ± 0.14 mL versus 0.43 ± 0.10 mL versus 0.40 ± 0.11 Ml; p-value = 0.3260) and the left ventricle final diastolic volume (0.63 ± 0.15 mL versus 0.56 ± 0.14 mL versus 0.54 ± 0.13 mL; p-value = 0.1891) did not present significant differences in the pre-transplantation period in the Control, Mononuclear and Mesenchymal groups, respectively. The three groups were considered homogenous.
One month after the transplantation, the LVEF presented a significant decrease in the Control Group (26.84 ± 7.05 to 22.32 ± 6.94%; p-value = 0.0045) but it remained stable in the Mononuclear and Mesenchymal Groups (21.79 ± 8.77% to 18.60 ± 6.11%; p-value = 0.4232 and 26.62 ± 7.34% to 25.55 ± 10.21%; p-value = 0.6505, respectively) - Table 1.
The LVFSV over the same time interval gave significant increases in the Control Group (0.46 ± 0.14 mL to 0.60 ± 0.17 mL; p-value = 0.0001), in the Mononuclear Group (0.43 ± 0.10 mL to 0.77 ± 0.13 mL; p-value = 0.0002) and the Mesenchymal Group (0.40 ± 0.11 mL to 0.52 ± 0.18 mL; p-value = 0.0003) - Table 2.
In LVFDV over the same time interval gave significant increases in the Control Group (0.63 ± 0.15 mL to 0.76 ± 0.17 mL; p-value = 0.0013), in the Mononuclear Group (0.56 ± 0.14 mL to 0.95 ± 0.18 mL; p-value = 0.0003) and the Mesenchymal Group (0.54 ± 0.13 mL to 0.69 ± 0.17 mL; p-value < 0.0001).
Analysis among groups
After one month from cell transplantation no significant statistical differences were identified in respect to the LVEF among the Control, Mononuclear and Mesenchymal Groups (22.32 ± 6.94% versus 18.60 ± 6.11% versus 25.55 ± 10.21%, respectively; p-value = 0.2980). Over this same time interval, significant statistical differences between control, mononuclear and mesenchymal groups were identified in respect to the LVFSV (0.60 ± 0.17 mL versus 0.77 ± 0.13 mL versus 0.52 ± 0.18 Ml; p-value = 0.0005) and LVFDV (0.76 ± 0.17 mL versus 0.95 ± 0.18 mL versus 0.69 ± 0.17 Ml; p-value = 0.002).
Histology
Histological analysis of the Control Group identified a great quantity of fibrosis but no evidence of the formation of any other type of tissue (Figure 2).
Analysis of Mononuclear Group identified the presence of neovessels and endothelial cells in the fibrotic region (Figures 3 and 4).
An analysis of the Mesenchymal Group identified the presence of neovessels, endothelial cells and smooth muscle cells (Figures 5 and 6).
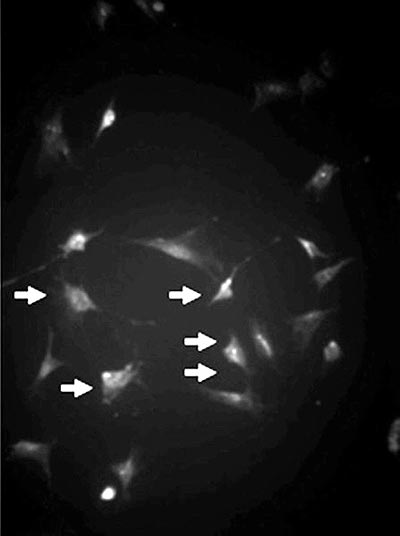
Fig. 1 - Mesenchymal cell culture (arrows) identified by the anti-vimentin antibody (Immunofluorescence, x 200)
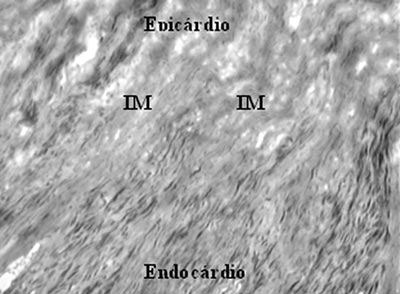
Fig. 2 - Myocardial infarction (MI) - Gomori's Trichrome x 400
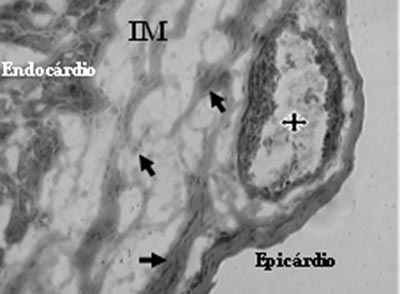
Fig. 3 - Neovessels with lumen (stars) and endothelial cells (arrows) after mononuclear cell transplantation in myocardial infarction (MI) - Gomori's Trichrome x 200
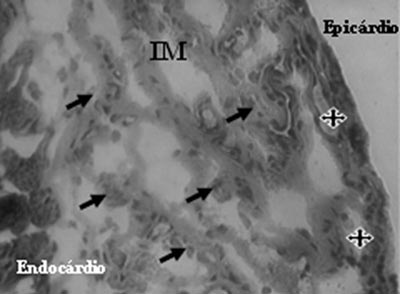
Fig. 4 - Endothelial cells (arrows) and neovessels (stars) identified after mononuclear cell transplantation in myocardial infarction (MI) - Gomori's Trichrome, x 200
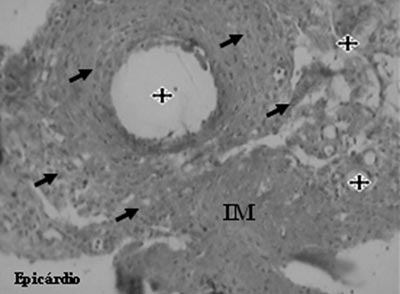
Fig. 5 - Neovessels (stars) and endothelial cells (arrows) after mesenchymal cell transplantation in myocardial infarction (MI - Gomori's Trichrome, x 200
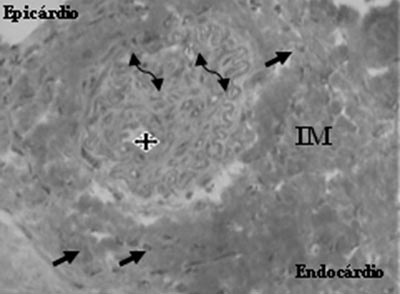
Fig. 6 - Neovessel (star), endothelial cells (arrows) and smooth muscle cells (double arrows), after the transplantation of mesenchymal cells in myocardial infarction (MI) - Gomori's Trichrome, x 400
COMMENTS
The main goal of cell transplantation in infarcted myocardium is the colonization of the fibrotic region with new contractile cells to recover the left ventricle contractile function. In our study, in a model of chronic myocardial infarction with pre-established fibrosis, we identified that bone marrow cells both the mononuclear cells and the mesenchymal cells, presented similar results however, some differences should be discussed.
Bone marrow cell transplantation in the myocardium has presented distinct results in several works, both experimental and clinical. It is important to remember that different cell fractions exist in the bone marrow being utilized in myocardial therapy (mononuclear, mesenchymal and hematopoietic cells), as there are also different models of myocardial disease, where the cells are being transplanted (transmural fibrosis, myocardial ischemia and hibernated myocardium) and, consequently different functional, clinical and anatomopathological results are identified.
There are authors who recommend only the mobilization of circulating bone marrow cells, stimulated by the G-CSF. The results suggest the power of recovery of the infarcted myocardium [16].
In regards to mononuclear cell transplantation, Orlic et al. [17] demonstrated the benefits of cell transplantation in the infarcted myocardium transition zone and the intact myocardium, after coronary artery occlusion. They reported that part of the infarcted region was colonized by new tissues: cardiomyocytes and blood vessels. In this study, the bone marrow cells were transplanted into a region which still had viable heart muscle, because the mononuclear cells were transplanted into the transition zone between the intact myocardium and the infarcted myocardium, where hibernating myocardium exists; it is comprehensible that the transplanted cells can differentiate into cardiomyocytes.
On the other hand, Bel et al. [12] injected mononuclear cells in a myocardial infarction model with pre-established fibrosis. Thirty days after cell transplantation, deterioration of the ventricular function was identified, both in the Control Group and in the group that received the cells. In regards to the histological analysis, no new contractile tissue was identified in the myocardial fibrosis, just a higher level of macrophages and new blood vessels. Thus, the authors recommend caution in respect to the real benefits of bone marrow cell transplantation.
An important clinical study with bone marrow cells was performed by Wollert et al. [18], in which patients were submitted to the treatment of acute myocardial infarction by intracoronary angioplasty and after, two distinct treatments: medicinal therapy and bone marrow cell transplantation. The authors suggest significant functional improvement in the group that received bone marrow cells when compared to the group that received clinical treatment. These results are very interesting however they must be analyzed carefully, as the article refers to the treatment of acute myocardial infarction by intracoronary angioplasty and not isolated bone marrow cell transplantation. The benefit of bone marrow cell transplantation is better when compared to the clinical treatment after intracoronary angioplasty however, both groups presented clinical improvements after the intracoronary angioplasty and not only the group treated with bone marrow cells. Physiologically it was not the bone marrow cell transplantation alone that promoted the functional improvement of the heart, but it was the intracoronary angioplasty.
Another study [13] in which the results of bone marrow cell and skeletal myoblasts transplantation were compared in a model of myocardial infarction with transmural fibrosis, functional improvement of the group that received skeletal cells was identified however, the group that received bone marrow cells presented a stabilization of the function. A histological evaluation identified the presence of new skeletal-type fibers in the Myoblast Group and new blood vessels in the group that received bone marrow cells, which justifies the lack of functional improvement in this group. However, stabilization of its function and the fact that it did not worsen can be explained because, differently of myoblasts, bone marrow cells may exert mechanisms that limit the progression of the infarction, probably by neoangiogenesis.
In our study, in respect to the functional analysis in the pre-transplantation period, the three groups presented with ejection fractions and left ventricle final systolic and diastolic volumes without exhibiting statistically significant differences among them, confirming the homogeneity of groups. The experimental model utilized was the one of myocardial infarction with pre-established fibrosis and severe ventricular dysfunction, as the animals included in the study presented mean left ventricle ejection fractions of less than 27%.
The Control Group presented a significant deterioration in the left ventricle ejection fraction thirty days after myocardial infarction, with a p-value = 0.0045, confirming the effect of the muscle necrosis and consequently cardiac insufficiency, as was expected. Both the group that received mononuclear cells and the other group that received mesenchymal cells, presented with similar results, in spite of not presenting improvements in the left ventricle ejection fraction, both presented functional stabilization, with p-values = 0.4232 and 0.6505, respectively, between the period immediately after infarction and thirty days after cell transplantation.
In respect to the left ventricle final diastolic volume, the three studied groups presented increases thirty days after the injection of the cells. In the Control Group, because of the deterioration of the post-infarction ventricular function, enlargement and ventricular remodeling were already expected. However, in relation to the groups who received cell transplantation, despite of the stabilization of the left ventricle ejection fraction, enlargement and ventricular remodeling were also identified over this same period.
The left ventricle final systolic volume for all three groups studied presented an increase over the same time interval, suggesting a loss of the contractile capacity in all animals submitted to the study. As in the analysis of the final diastolic volume, the increase in the left ventricle final systolic volume in the Control Group was expected because of the evolution of myocardial infarction, but these data were also similar in the groups that underwent cell transplantation.
In respect to the increase of ventricular volumes (LVFDV and LVFSV), even after cell transplantation, it is believed that this effect is directly related to the natural evolution of the underlying disease. As only animals with ejection fractions of less than 30% were included in the study and as there was a severe ventricular dysfunction with increases in ventricular volumes, previous to transplantation, it is difficult to understand how the transplantation of cells can exert an anti-remodeling mechanism, as the treatment is only local. Moreover, in the pathological examination, only new blood vessels were identified in the region of infarction where the cells had been transplanted.
Thus, despite the limiting mechanism of the progression of the infarction after cell therapy proposed by Garot et al. [19], therapy with bone marrow cells (both mononuclear and mesenchymal) in chronic myocardial infarction in our study did not exert the anti-remodeling effect. A justification for this is that the transplantation of both types of cells was performed in the left ventricle anterior wall, so their effect was only local. Another explanation for the ventricular remodeling, identified in all animals that received cells, may be related to the experimental study model utilized, as the animals that were submitted to transplantation had presented with pre-existent severe ventricular dysfunction, with increases in the ventricular volumes. Some studies performing bone marrow cells transplantation in hearts without severe ventricular dysfunction may present results different to those found in our study.
In respect to the pathological examination, the analysis was only morphological and comparative among the groups that received cells and the Control Group. In the myocardial infarction region, endothelial cells were identified in both groups that received cells. In the group that received mesenchymal cells, as well as the endothelial cells smooth muscle cells were also identified, which are part of the newly-formed vascular structure. Striated muscle fibers were not identified in the infarcted region where the cells were transplanted. The Control Group presented only transmural fibrosis. Thus, it is understood that the lack of functional improvement of groups where the cell transplantations were performed is due to the fact that new striated contractile cells were not identified in the infarcted region.
The mechanisms that can explain why groups that received mononuclear and mesenchymal cells presented stabilization of the left ventricle ejection fraction are based on the presence of angiogenesis identified in the transplanted region. These newly-formed vessels can increase the blood supply to the transition zone between the infarcted and the intact myocardium, elevating the contractility of hibernated cells and also preventing apoptosis. Some authors still believe that the mononuclear bone marrow cells present an anti-inflammatory effect, identified in animals with Chagas disease [20] and others suggest that the bone marrow cells can stimulate the production of cytoprotector proteins (HsPS, HsP32, HsP70 and VEGF) [21].
Silva et al. [22] only identified an improvement of the myocardial perfusion in animals with severe ventricular dysfunction in a myocardial ischemia model, as did Bel et al. [12], in animals with severe ventricular dysfunction in a myocardial infarction model with fibrosis. Thompson et al. [23], also using a myocardial infarction model with fibrosis, did not identify cardiomyocytes in the region of myocardial necrosis in a group that received mononuclear bone marrow cells, the cardiomyocytes were only located in the transition zone between the intact and infarcted myocardium.
One of the most important functional results after mononuclear cell transplantation was reported by Marzullo [24], who analyzed a region of myocardial fibrosis two times using myocardial scintigraphy: one of perfusion and one of myocardial contraction. In the myocardial perfusion analysis, an increase of the blood irrigation was identified at the site where the cells were transplanted, while when the myocardial contraction was analyzed this result was not observed. This suggests that bone marrow cell transplantation in transmural myocardial fibrosis can improve the perfusion, but not the myocardial contraction.
Remembering that bone marrow cells have a characteristic of slightly-dependent differentiation [8], how can we expect that bone marrow cells can differentiate into cardiomyocytes in transmural areas of myocardial fibrosis? On the other hand, when the bone marrow cells are injected in a myocardial ischemia model, with cells that are still viable or in transition zones between the myocardial infarction and the intact myocardium or, even, where there is the presence of intermixed myocardial fibrosis with intact myocardium, this trans-differentiation is justifiable and comprehensible.
In respect to some differences between the functional and anatomopathological results of our study and some other published studies, it is important to differentiate that the type of study model utilized in our study was a chronic myocardial infarction model with pre-established fibrosis, different to other authors, who utilized acute infarction [25], myocardial ischemia [22] or hibernated myocardium [23]. It is known that the mechanism of myocardial regeneration from acute, chronic and ischemic myocardial infarction are distinct, thus, the results are too.
In respect to the isolation of the different fractions of bone marrow cells, mononuclear cells are obtained after a simpler process than the mesenchymal cells, because they need only to pass through two processes of centrifugation and through the Ficoll-Hypaque density gradient (Boyum method) [26], while the mesenchymal cells need to pass through these same processes and after through cell culture and cell expansion for approximately 14 to 16 days, presenting much higher costs and a greater risk of contamination.
The functional results of the mononuclear and mesenchymal cells were superimposed on the evaluated parameters. In relation to the pathological examination, both presented with neoangiogenesis, however, the process was a little more complete in the group that received mesenchymal cells seen by the presence of smooth muscle cells.
As the main characteristic of the bone marrow cells is neoangiogenesis, we believe that this may be an excellent option for the treatment of hibernated myocardium that does not have the technical conditions for myocardial revascularization surgery or coronary angioplasty and also for patients resistant to the medicinal clinical treatment.
CONCLUSION
The data identified in this study suggest that both the group that received mononuclear cells and the group that received mesenchymal cells presented with a homogenous stabilization of the left ventricle ejection fraction and vascular regeneration, but without the ventricular anti-remodeling effect when transplanted in the myocardial infarction.
ACKNOWLEDGEMENTS
The authors wish to thank CAPES for their support.
BIBLIOGRAPHIC REFERENCES
1. Kajstura J, Leri A, Finato N, Di Loreto C, Beltrami CA, Anversa P. Myocyte proliferation in end-stage cardiac failure in humans. Proc Natl Acad Sci USA. 1998;95(15):8801-5.
2. Ghostine S, Carrion C, Guarita-Souza LC, Bruneval P, Vilquin JT, Pouzet B et al. Long-term efficacy of myoblast transplantation on regional structure and function after myocardium infarction. Circulation. 2002;106(12 suppl. l):I-131-6.
3. Guarita-Souza LC, Carvalho RG, Pouzet B, Vilquin JT, Garcin I, Menasché P et al. The transplant of cardiac cells and myoblast skeletal cells in myocardial infarction. Rev Bras Cir Cardiovasc. 2002;17(4):312-22.
4. Murry CE, Wiseman RW, Schwartz SM, Hauschka SD. Skeletal myoblast transplantation for repair of myocardial necrosis. J Clin Invest. 1996;98(11):2512-23.
5. Scorsin M, Hagège A, Vilquin JT, Fiszman M, Marotte F, Samuel JL et al. Comparison of the effects of fetal cardiomyocyte and skeletal myoblast transplantation on post-infarction left ventricular function. J Thorac Cardiovasc Surg. 2000;119(6):1169-75.
6. Taylor DA, Atkins BZ, Hungspreugs P, Jones TR, Reedy MC, Hutcheson KA et al. Regenerating functional myocardium: improved performance after skeletal myoblast transplantation. Nat Med. 1998;4(8):929-33.
7. Menasche P, Hagege AA, Vilquin JT, Desnos A, Abergel E, Pouzet B et al. Autologous skeletal myoblast transplantation for severe post-infarction left ventricular dysfunction. J Am Coll Cardiol. 2003;41(7):1078-83.
8. Verfailie C.M. Adult stem cells: assessing the case for pluripotency. Trends Cells Biol. 2002;12(11):502-8.
9. Friedenstein AJ, Petrakova KV, Kurolesova AI, Frolova GP. Heterotopic of bone marrow: analysis of precursor cells for osteogenic and hematopoietic tissues. Transplantation. 1968;6(2):230-47.
10. Wang JA, Fan YQ, Li CL, He H, Sun Y, Lv BJ et al. Human bone marrow-derived mesenchymal stem cells transplanted into damaged rabbit heart to improve heart function. J Zhejiang Univ Sci B. 2005;6(4):242-8.
11. Deb A, Wang S, Skelding KA, Miller D, Simper D, Caplice NM et al. Bone marrow-derived cardiomyocytes are present in adult human heart: a study of gender-mismatched bone marrow transplantation patients. Circulation. 2003;107(9):1247-9.
12. Bel A, Messas E, Agbulut O, Richard P, Samuel JL, Bruneval P et al. Transplantation of autologous fresh bone marrow into infarcted myocardium: a word of caution. Circulation. 2003;108(Suppl 1):II247-52.
13. Guarita-Souza LC, Carvalho K., Rebelatto C, Miyague N, Furuta M, Scorsin M et al Effectiveness of skeletal myoblasts and bone marrow stromal cells transplantation on post-infarct ventricular dysfunction. Circulation. 2003;108(17), Suppl: IV-218.
14. Agbulut O, Vandervelde S, Al Attar N, Larghero J, Ghostine S, Leobon B et al. Comparison of human skeletal myoblasts and bone marrow-derived CD133+ progenitors for the repair of infarcted myocardium. J Am Coll Cardiol. 2004;44(2):458-63.
15. Perin EC, Dohmann HF, Borojevic R, Silva SA, Souza AL, Mesquita CT et al. Transendocardial, autologous bone marrow cell transplantation for severe, chronic ischemic heart failure. Circulation. 2003;107(18):2294-302.
16. Orlic D, Kajstura J, Chimenti S, Limana F, Jakoniuk I, Quaini F et al. Mobilized bone marrow cells repair the infarcted heart improving function and survival. Proc Natl Acad Sci USA. 2001;98(18):10344-9.
17. Orlic D, Kajstura J, Chimenti S, Jakoniuk I, Anderson SM, Li B et al. Bone marrow cells regenerate infarcted myocardium. Nature. 2001;410(6829):701-5.
18. Wollert KC, Meyer GP, Lotz J, Ringes-Lichtenberg S, Lippolt P, Breidenbach C et al. Intracoronary autologous bone-marrow cell transfer after myocardial infarction: the BOOST randomised controlled clinical trial. Lancet. 2004;364(9429):141-8.
19. Garot J, Unterseeh T, Teiger E, Champagne S, Chazaud B, Gherardi R et al. Magnetic resonance imaging of targeted catheter-based implantation of myogenic precursor cells into infarcted left ventricular myocardium. J Am Coll Cardiol. 2003;41(10):1841-6.
20. Soares MB, Lima RS, Rocha LL, Takyia CM, Carvalho AC, Ribeiro-dos-Santos R et al. Transplanted bone marrow cells repair heart tissue and reduce myocarditis in chronic chagasic mice. Am J Pathol. 2004;164(2):441-7.
21. Penn MS, Francis GS, Ellis SG, Young JB, McCarthy PM, Topol EJ. Autologous cell transplantation for the treatment of damaged myocardium. Prog Cardiovasc Dis. 2002;45(1):21-32.
22. Silva GV, Litovsky S, Assad JA, Sousa AL, Martin BJ, Vela D et al. Mesenchymal stem cells differentiate into an endothelial phenotype, enhance vascular density, and improve heart function in a canine chronic ischemia model. Circulation. 2005;111(2):150-6.
23. Thompson RB, Emani SM, Davis BH, Van den Bos EJ, Morimoto Y, Craig D et al. Comparison of intracardiac cell transplantation: autologous skeletal myoblasts versus bone marrow cells. Circulation. 2003;108(suppl 1):II-264-71.
24. Marzullo P. Nuclear imaging after cell implantation. Int J Cardiol. 2004;Supll 1, S53-S54.
25. Orlic D, Kajstura J, Chimenti S, Bodine DM, Leri A, Anversa P. Transplanted adult bone marrow cells repair myocardial infarcts in mice. Ann N Y Acad Sci. 2001;938:221-9.
26. Boyüm A. Isolation of mononuclear cells and granulocytes from human blood: isolation of mononuclear cells by one centrifugation and of granulocytes by combining centrifugation and sedimentation at 1g. Scand J Clin Lab Invest Suppl. 1968;97:77-89.