Objective: To evaluate if the use of hemostatic high-dose aprotinin seems influence to myocardial, renal and metabolic functions in children submitted to surgical correction with extracorporeal circulation (ECC). Material and Methods: A prospective randomized study was conducted on children aged 30 days to 4 years submitted to correction of acyanogenic congenital heart disease with ECC and divided into two groups: Control (n=9) and Aprotinin (n=10). In the Aprotinin Group the drug was administered before and during ECC and the myocardial and multiorgan dysfunctions were analyzed on the basis of clinical and biochemical markers. Differences were considered to be significant when P<0.05. Results: The groups were similar regarding demographic and intraoperative variables, except for a greater hemodilution in the Aprotinin Group. The drug had no benefit regarding time of mechanical pulmonary ventilation, permanence in the pediatric postoperative intensive care unit (ICU) and length of hospitalization, or regarding the use of inotropic drugs and renal function. The partial arterial oxygen pressure/inspired oxygen fraction ratio (PaO2/FiO2) was significantly reduced 24h after surgery in the Control Group. Blood loss was similar for both groups. Cardiac troponin I (cTnI), creatine kinase MB fraction (CKMB), serum glutamic-oxaloacetic transaminase (SGOT) and the aminoterminal fraction of natriuretic peptide type B (NT-proBNP) did not differ significantly between groups. Post-ECC blood lactate concentration and metabolic acidosis was more intense in the Aprotinin Group. There were no complications with the use of aprotinin. Conclusion: High-dose aprotinin did not significant influence in serum markers troponin I, NT-proBNP and renal function, but did associated with hemodilution, blood lactate concentration and metabolic acidosis more intense.
Objetivo: Avaliar se o uso de aprotinina em altas doses hemostáticas pode influenciar as funções miocárdicas, renais e metabólicas em crianças operadas com circulação extracorpórea (CEC). Métodos: Estudo prospectivo randomizado em crianças de 30 dias a 4 anos de idade, submetidas à correção de cardiopatia congênita acianogênica, com CEC e divididas em dois grupos, um denominado Controle (n=9) e o outro, Aprotinina (n=10). Neste, a droga foi administrada antes e durante a CEC. As disfunções miocárdicas e multiorgânicas foram analisadas por marcadores clínicos e bioquímicos. Foram consideradas significantes as diferenças com P<0,05. Resultados: Os grupos foram semelhantes quanto às variáveis demográficas e intra-operatórias, exceto por maior hemodiluição no Grupo Aprotinina. Não houve benefício quanto aos tempos de ventilação pulmonar mecânica, permanência no Centro de Terapia Intensiva Pediátrica (CTIP) e hospitalar, nem quanto ao uso de inotrópicos e função renal. A relação PaO2/FiO2 (pressão parcial de oxigênio arterial/fração inspirada de oxigênio) apresentou queda significativa com 24h PO, no Grupo Controle. As perdas sanguíneas foram semelhantes nos dois grupos. Os marcadores troponina I cardíaca (cTnI), fração MB da creatinofosfoquinase (CKMB), transaminase glutâmico-oxalacética (TGO) e fração amino-terminal do peptídio natriurético tipo B (NT-proBNP) não apresentaram diferenças marcantes inter-grupos. A lactatemia e acidose metabólica pós-CEC foi maior no Grupo Aprotinina. Não houve complicações tromboembólicas, neurológicas ou de hipersensibilidade com o uso da aprotinina. Conclusão: A aprotinina em altas doses não influenciou significativamente nos marcadores séricos troponina I e NT-proBNP e de função renal, porém foi associado com maior hemodiluição, lactatemia e acidose metabólica.
INTRODUCTION
The surgical techniques and the perioperative multidiscipline approach used in children holding congenital cardiopathies have presented important advances in the last few years, however, still with important risks of post-perfusion multiorganic systemic dysfunction, expressed by myocardial depression, vasomotor dysfunction, respiratory, renal or hepatic insufficiencies, neurocognitive and thermal regulation maladjustments, and bleeding by coagulopathy (systemic inflammatory response syndrome - SIRS), triggered by the limited biocompatibility of the materials during extracorporeal circulation (ECC). These factors might determine the exposition to a higher number of hemoderivative donors, prolonged time of hemostasia in the operation room, need of late sternal closure, with consequent prolongation of mechanical ventilation and stay in the Pediatric Intensive Care Unit (PICU), and preoccupying morbidity-mortality associated to high hospital expenses [1-4].
The use of aprotinin, an unspecific inhibitor of serine proteases consisted of a hydrophilic and basic polypeptide chain of 6512 Daltons, which haemostatic property reduces bleeding after ECC in children, was one of the medicine strategies used to prevent, avoid or fight coagulopathy and the frequent referred complications in this population [1].
The haemostatic property of aprotinin expressed by the reduction in bleeding after ECC was discovered casually at the Hammersmith Hospital, due to a study investigating its anti-inflammatory role with a high dose denominated dosis, measured in kalicreine inhibiting units (CIU). It was observed that, although plasmatic concentrations of, approximately, 50 to 125 CIU/ml (approximately 1 µM) do inhibit plasmine; in order to inhibit calicreine, coagulating agents and neutrophilic elastase they require higher concentrations, of 200 to 400 CIU/ml (approximately 4 - 8 µM) [1]. Even much higher doses seem to be well tolerated, once there were no side effects in poly-traumatized patients treated with 17.5 millions CIU of the drug, in a 24h period [5].
In the haemostatic doses, there were reported anti-inflammatory effects of aprotinin [1], alleviating the lesion of ischemia-reperfusion in deferent organs, including the heart [6]. The preservation and/or recuperation of the myocardial function treated by high dosage of aprotinin, and expressed by less inotropic support in the first 48h after ECC, was shown by Wippermann et al. [7], in 1999. On the other hand, Broche et al. [8], in 2002, reported a decrease in the production of free radicals and systemic oxidative stress, by aprotinin, in children under mechanical cardio-respiratory support, with secondary reduction of the low debt cardiac syndrome and arrhythmias, whilst Mössinger et al. [2], in 2003, showed that the drug reduces pulmonary and haemostatic dysfunction levels.
It had not been observed the incidence of myocardial infarction (IMA), acute renal insufficiency (ARI) and cerebrovascular accident (CVA) with aprotinin in adults, until a non-randomized observational study reported the contrary [9], also establishing higher mortality 5 years after surgery. With the results of the Canadian BART study (
Blood conservation using Anti-fibrinolytics: a Randomized Trial in a cardiac surgery population study), suggesting increase in the risk of death [10], the production of aprotinin was suspended in November of 2007.
The pediatric studies with aprotinin fail for their difficult interpretation, due to the vast variation in dosis, the large variability of the metabolism and antifibrinolytic action of the aprotinin in neonates, hemodilution, dispersion on the coots ages studied in the several studies and the different surgical interventions performed with consequent variability in the ECC time and type of surgery [2,3]. There is no sufficient data to affirm that aprotinin is safe and efficient in children[4], however, out of 36 member centers of the
Pediatric Cardiac Intensive Care Society (22 of them located in the United States), 83% employ the drug in pediatric cardiac surgery routinely, with ECC [11].
There are no studies proving the toxicity and influence in morbidity and mortality of high haemostatic doses of aprotinin in children operated with ECC, especially in the most susceptible population of neonates and lactents. There were not observed any higher risks of thromboembolic events or, more recently, association with renal dysfunction, dialysis, neurological complications and mortality in children using aprotinin [1,3,12-14].
It was also questioned whether the use of aprotinin in pediatric cardiac surgery should be suspended for the drug association to adverse effects in adults. The application of security data in adults for the pediatric practice demands caution and its validity is questionable, for they are two distinctive populations, with different risk factors, and drugs that present higher risk of complications in adults might be safe in children, and vice-versa. The perspectives for future randomized and controlled pediatric studies with aprotinin seem to be limited at the moment [4,15].
Based on the exposed data, noting the medical and legal implications of the temporary suspension in production of aprotinin, along with the fact of no reports stating significant adverse effects in children, especially renal effects, and lacking available information on the metabolic, myocardial and neuro-humoral effects of high doses of Aprotinin, when used with haemostatic purpose, which still arises scientific interest, the present work was performed in the attempt of showing these possible effects in a subgroup of children with acyanogenic congenital heart disease operated by our staff.
Objective
Verify the myocardial, renal and metabolic effects of the intraoperative administration of high haemostatic doses of aprotinin in children submitted to correction of acyanogenic congenital heart disease with ECC.
METHODS
Patients
There were studied 19 children, of both genders, submitted to correction of acyanogenic congenital heart disease with ECC, from January to December, 2004. The patients were distributed, randomly, in two groups: Aprotinin (n = 10) and Control (n = 9). The study was clinical, perspective, randomized and not blind. The criteria of inclusion were elective operations and age range between one month and four years, and the exclusion criteria: previous cardiovascular operation, exposition to aprotinin six months prior to surgery; use of salicylates until seven days before operation; allergic-immunologic, hepatic, renal or coagulation disturbances and episodes of cardiac arrest, sepsis and vasculitis less than two months prior to surgery.
The parents or legal tutors signed up consent term. The study was approved by the Ethic Research in Human Beings Committee of HC FMRP-USP, under the number # 6665/2004.
Methodology
The patient distribution, in both groups, was randomized, after anesthetic induction.
Anesthetic technique
In the pre-anesthesia room midazolan was administered. In the operation room, children laying down on a thermic mattress and under warm air flow were monitored with electrocardiogram (ECG) and oxymetry. The anesthetic induction consisted of endovenous injections (EV) of midazolan, fentanil or sulfentanil, and muscular relaxation, with vecuronium or pancuronium. The anesthetic plan was carried out with fentanil or sulfentanil associated to isofluorane inhaling, oral-tracheal intubation and mechanical pulmonary ventilation. The invasive monitoring of blood pressure (BP) was performed by radial or femoral artery punction. The right internal jugular vein or the femoral vein was cannulated liquid infusion and the central venous pressure control (CVPC) and the oral-pharynx and peripheral temperatures (foot sole) were recorded. Diuresis was measured. The rachideal anesthesia, with morphine and clonidine, was performed by the anesthesia crew (three patients of each group). It was avoided the use of corticosteroid. After anesthetic induction, it was administered amicacine 7.5 mg/kg EV, associated to cefazoline (40 mg/kg EV) in the children interned less than 48h. On the rest, the association was performed with vancomicine (10 mg/kg EV). An additional dose of vancomicine (5 mg/kg) was added in the oxygenator reservoir. Aprotinin (Trasylol®, Bayer, Leverkusen, Germany), dosage of 240mg/m² EV, was applied 20 to 30 min, beginning the surgery, followed by continuous infusion of aprotinin, 56mg/m²/h until the curative procedures after surgery. The drug (240mg/m²) was also added to the priming of the oxygenator. Ten minutes prior to the aprotinin infusion, a sensitivity test was performed with a small dose of 10.000 CIU EV.
Surgical technique and ECC
Under topical antisepsis with iodide polivinilpirrolidone; medium sternotomy and total timectomy were performed. The children were heparinized (3mg/kg EV) (Sodium Heparin, Roche, Basle, Switzerland), under controlled activated coagulation time (ACT) (Hemotec ACT II®, Medtronic, Englewood, CO, U.S.A.), maintaining ACT above 480s, in both groups (with additional heparin doses of 0.5 mg/kg EV). The ascending aorta and the vena-cavas were cannulated separately. In the ECC, performed with passive venous draining, using four similar types of membrane oxygenators, especially Polystan Safe Mini or Micro (MAQUET Gmbh & Co, KG, Medical Systems Com. Ind. Medica Ltda., SP, Brazil) or D901 Lilliput 1 DIDECO (Cobe CV, Sorin Group Company, Mirandola, Italy), with the respective arterial filters. There were used roller pumps, with blood flow, in normothermia, of 2.5 l/m²/min. The oral-pharynx temperature, in ECC, was reduced to 28°C, by thermopermutation, in the oxygenator.
The primming was calculated in order to result with hematocryt at 30% and consisted of a concentration of erythrocytes, Ringer solution, frozen fresh plasma, mannitol at 20% (4 to 5ml/kg), sodium heparin (Roche Pharma, Basle, Switzerland) 1mg/10 ml of hemoderivative and sodium bicarbonate at 8.4% 1mEq/kg. In the Aprotinin Group, the volume of the drug added to the primming was included in the calculation. Hemoconcentrators with poliariletersulfone membrane were used for ultrafiltration, started in the reheating. The hematocryt during the ECC was maintained by addition of a concentration of erythrocytes. After the aortic clamping, the hyperkalemic anterograde cardioplegia (10 ml/kg), at 4°C, hoisted at one meter high from the operation table, was passively infused, at the aortic root and repeated every 30 min. The first dose was of crystalloid and the rest of blood. The systemic reheating, up to 37°C, was started simultaneously with the infusion (0.5 to 1.5 µg/kg/min) of sodium nitroprussiate. At the end of the ECC, the aortic and superior vena cava cannulas were removed, and the arterial line was connected to the inferior vena cava cannula.
The volume present in the oxygenator started to be ultrafiltrated, in the intervals between refilling of volume, infused by the inferior vena cava cannula. It was administered protamine cloridrate (ICN Pharmaceuticals Ltd., Valeant Pharmaceuticals International, U.S.A.), proportion of 1:1, according to the total dose of heparin used, and it was confirmed the return of ACT to basal levels. The left-over blood in the ECC circuit was retrieved in transfer bags without anticoagulant for infusion EV by venous catheter after removing the cannula. The pericardium was closed, in case it had no hemodynamic instability. A mediastinal drain was implanted, under aspiration of 20 cm of water. Regular synthesis.
Preoperative clinical characteristics
There were analyzed the demographic variables (age, gender, weight, height and body surface) and calculated the risk categories according the scores of The
Risk Adjusted Classification for Congenital Heart Surgery (RACHS-1) and Basic Aristotle, as well as Ross and Reithmann, modified, to congestive cardiac insufficiency (CCI). The medications in use, the presence of arrhythmias, ECG, thoracic radiography, ecoDopplercardiogram, cardiac catheterism and surgical diagnosis were recorded, and the hemodynamic variables filed (pulmonary and systemic blood pressure), and acquired hemogram, coagulogram and renal function tests (plasmatic urea and creatinine) and hepatic (glutamic-oxalacetic transaminase - GOT and bilirubins).
Surgical data
There were collected data as performed operation, intracardiac access ways, duration of surgery, anesthesia and ECC, aortic clamping time, minimum oral-pharynx temperature, hydric balance, diuresis, volume of erythrocytes concentration, fresh plasma and platelet concentration, TCA antes, during and after ECC and complications.
Postoperative clinical conditions
Patients were treated according the protocol of postoperative treatment. After arriving at the PICU, it was calculated the PRISM score (
Pediatric Risk Index Score for Mortality). The inotropic scores I and II [16,17] (Chart 1) were verified the following 48h.
Retrospectively, it was calculated the index recently proposed by Mattos et al. [18], in 2006. The time of usage of inotropics, vasoactive drugs EV, nitric oxide, and stay in PICU and the time elapsed until hospital release or death were measured, as well as the duration of mechanical pulmonary ventilation (MV). Postoperative bleeding (PO) accumulated at 4, 12, 24 and 48h, and the use of hemoderivatives, at 6 and 24h of PO, were expressed in ml/kg, and the number of donors to which patients were exposed was recorded. Renal dysfunction was evaluated by the volume of diuresis, dosage of plasmatic urea and plasmatic creatinine and depuration of creatinine estimated by the Schwartz formula. Hemodynamic, respiratory and metabolic aspects, coma scale of Glasgow and hepatic dysfunction were recorded [19], as well as the PO complications.
Biochemical and hematological evaluation
Arterial blood samples (3 ml) were collected in the following moments: T1 - After anesthesia induction, before the administration of Aprotinin; T2 - 15 min after starting ECC; T3 - Immediately before the end of ECC; T4 - 5 min after administration of protamine; T5 - 4 h after T4; T6 - 12 h after T4; T7 - 24 h after T4; T8 - 48 h after T4. The concentrations of ions Na, K, Ca, de urea, creatinine, fraction of creatine kinase MB (CKMB), GOT and bilirubins, as well as the times of protrombine (TP) by INR (
International Normalized Ratio) and partial activated tromboplastine (PAT) were measured. The rates of hemoglobin and hematocryt, count of leukocytes and platelets were verified in times T1 to T8. The gasometry and the serum lactate were determined in the arterial blood (analyzer Rapilab 860, Bayer AG, Leverkusen, Germany), in times T1 to T8. Three ml of blood of each sample were preserved in tubes without anticoagulant, with separating gel (BD Vacutainer Systems®, Belliver Industrial State, Plymouth, United Kingdom ) at 4°C, and, afterwards, centrifuged at the same temperature (10 min, at 3000 rpm).
The fluid obtained was pipetted and stored at a temperature of - 70°C, in tubes Eppendorf type, for later processing by immune-enzymatic essay (ELISA), for dosage of cardiac troponine I (cTnI) (IMMULITE®, Diagnostic Products Corporation, Los Angeles, CA, U.S.A.) in aliquots of 150µl or more of plasma. The analysis was performed at the Laboratory of Endocrinology of the HC-FMRP-USP. The fraction amino-terminal of the natriuretic peptide type B (Nt-proBNP) was dosed by enzymatic competitive immune-essay (ALPCO®, #catalog 04-BI-20852, Diagnostics Biomedical, Vienna, Austria), which reference values for healthy individuals: < 250 fmol/ml -negative result; < 250 - 350 fmol/ml - borderline result; > 350 fmol/ml -positive result. The kinetic profile of the serum levels cTnI and Nt-proBNP were quantified and corrected for hemodilution by serum albumin. The albumin was dosed in the plasma, by colorimetric method, in a spectrophotometer Bausch & Lomb (Model SPECTRONIC 70.1, U.S.A.); in times T1 to Tn (T2 to T4) (normal values 3.5 to 5.5 g/dl). The correction was performed using the following formula:

Statistical analysis
The qualitative variables were expressed in relative and absolute frequencies. The quantitative were expressed by minimum and maximum values, average, median and standard deviation. The intergroup comparison by analysis using a mixed model (package PROC MIXED®, program SAS/STAT®, version 9, SAS Institute Inc., Cary, NC, U.S.A.) presumed normal distribution, and was complemented by the correlation coefficient of Pearson (package PROC CORR®, programa SAS/STAT®, version 9, SAS Institute Inc., Cary, NC, U.S.A.). In the subgroup comparisons, as well as in the intragroup and intergroup comparisons, it was used a non-parametric exact test of Wilcoxon (program Software R Development Core Team (2005). R Foundation for Statistical Computing, Vienna, Austria. ISBN 3-900051-07-0, URL http://www.R-project.org). In the figures with boxes, the horizontal limits correspond to the 25º and 75º percentile, the line inside the box corresponds to the median and the external limits and lines of the boxes reflect the variable variance. The full points express the average, and the empty points, the discrepant values. The values of
P < 0.05 were considered statistically significant.
RESULTS
In the comparison between averages and standard deviations of the groups, those of the Aprotinin group will precede. In the Figures, the statistically significant intragroup differences, according to T1, are identified by *, and the intergroups, by**.
Preoperative
The groups were similar regarding age and anthropometric variables (Table 1). Except for the Ross score, modified, slightly accentuated in the Aprotinin group (median 5.5 vs. 3) (
P=0.56), the RACHS-1 (medium 2) (
P=0.11) and Basic Aristotle (median 6) (
P=0.82) were similar in both groups. The cardiac malformations were similar in both groups (Table 2), as well as the operations performed. The groups were considered statistically comparable.
Intraoperative
In the Aprotinin and Control groups, the duration of surgery (192.5 ± 39.3, median=185 min vs. 194.44 ± 35.30, median=210 min; P=0.87), anesthesia (319.5 ± 39.04, median=325 min vs. 288.33 ± 32.01, median=270 min; P=0.09), ECC (64 ± 21.05, median=62.50 vs. 69.44 ± 10.13, median=70 min; P=0.27), aortic clamping (39 ± 17.12, median= 36 min vs. 44.11 ± 7.11, median=45 min; P=0.13) oral-pharynx minimum temperature in ECC (28.9 ± 2.1, median = 28.7°C vs. 28.2 ± 2,7, median=29.1°C; P=0.81) presented no statistically significant differences. The oxygenators presented similar distribution in the groups. The total volume of priming and additions presented no statistically significant differences between groups (384 ± 185 ml vs. 430 ± 206 ml; P=0.45). The estimated volemia was similar in the groups (P=0.90). The total amount of aprotinin administered was 177.56 ± 77.76 mg, corresponding to 126.84 ± 55.55 ml of Trasylol. The total amount of heparin administered was similar between groups (39.2 ± 15.3 mg, median = 33.5 mg vs. 39.0 ± 16.7 mg, median = 30 mg), and corresponded to the mean values in the groups 7.4 ± 2,9 median = 7.3 vs. 7.1 ± 3.8 median = 6.6 mg/kg weight.
The use of concentration of erythrocytes, in ECC, (221 ± 55 ml vs. 248 ± 73 ml; P=0.64) and the hemofiltrated volume (378 ± 244 ml vs. 335 ± 319 ml) was similar (P>0.05). The diuresis (ml/kg) of both groups, before (4.60 ± 4.39 vs. 2.64 ± 2.64; P=0.41), during (19.22 ± 18.53 vs. 18.13 ± 17.81; P=0.82) and after (21.22 ± 31.64 vs. 11.93 ± 5.66, P=0.66) the ECC, was similar. The time of thoracic occlusion superposed (28.5 ± 9.1 min vs. 28.8 ± 17.4 min). Regarding the intraoperative blood balance, there was a negative balance in the Aprotinin Group, but with no significant difference between groups (-12 ± 166 ml, median=15 ml vs. 125 ± 81 ml, median=150 ml; P=0.05).
Both groups were fairly hypoalbuminemic, with similar basal rates (T1) (2.81 ± 0.38 g/dl vs. 2.81 ± 0.59 g/dl;
P=0.94). The ECC (T2) reduced abruptly this variable in both groups (1.32 ± 0.67 g/dl vs. 1.63 ± 0.47 g/dl;
P <0.01), up to the end. However, soon after the administration of protamine (T4), the albuminemia of the Aprotinin Group appeared statistically lower than the Control Group (
P=0.04) (Figure 1).
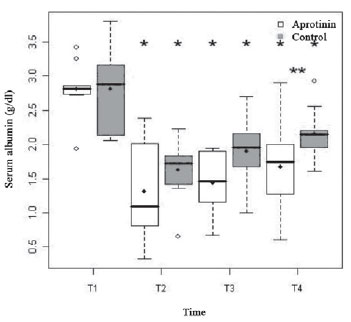
Fig. 1 - Albuminemia (g/dl) of the Aprotinin and Control Groups, in times T1 to T4
Postoperative
Regarding the hydric balance upon entering PICU, was similar in the groups (24 ± 77 ml/kg, median = 25 ml/kg vs. 31 ± 32 ml/kg, median= 35 ml/kg; P = 0.60). The PRISM score was worse in the Control Group (4.3 ± 3.4, median = 3 vs. 7.6 ± 4.2, median = 7), however, with no significant difference (P = 0.07). Both groups were similar regarding the clinical-surgical index of Mattos (5.3 ± 2.2, median = 5.5 vs. 4.7 ± 1.6, median = 5;
P > 0.05), confirming that both groups are homogeneous and comparable. 70% of the children of the Aprotinin Group and 88.8% of the Control Group were in the intermediary risk category (ages ranging from 1 month to 1 year). Protein-caloric malnutrition occurred in 90% vs. 77.7% of the children, nearly, high risk (below 5 percentile). Due to the presence of cardiac insufficiency, pulmonary high blood pressure and/or genetic syndrome (risk clinical factors associated), nearly half (50% vs. 55.5%) the children of both groups were high risk. All the children in the Control Group fit in the category of low risk regarding the surgical complexity (Basic Aristotle score), occurring in 80% of the Aprotinin Group. Regarding the time of ECC, all patients, except for one in the Aprotinin Group that exceeded 90 min, were of intermediary risk.
There were no significant differences between groups in the times using nitric oxide (1 case in aprotinin group and 2 cases in control group) (216 vs. 118 h), mechanical ventilation (79.50 ± 90.55, median 36 h vs. 79.77 ± 120.73 h, median 16h;
P = 0.81), stay in PICU (6.4 ± 4.92, median = 5.5 vs. 4.86 ± 4.80 days, median = 3 days;
P=0.27) and hospital stay (9.30 ± 4.99, median = 7.5 vs. 10.22 ± 11.68 days, median = 5 days). The groups were similar regarding the time of use of inotropics (122.40 ± 118.36 h, median = 132 h vs. 78.55 ± 89.40 h, median = 48 h;
P = 0.12). The higher inotropic scores occurred inT7 (score I - 4.5 ± 4 vs. 2.4 ± 2; score II - 23.5 ± 20 vs. 10.0 ± 5), higher in the Aprotinina Group, although with no difference statistically significant (
P=0.35 and
P=0.30, respectively).
In the Aprotinin and Control Groups, there was detected serious pulmonary congestion (4 vs. 3 patients), circulatory shock (5 vs. 3 patients), anasarca in 4 (two of them with ascites) vs. 2 patients and pleural effusions , two in each group. Both groups presented hyperthermia (37.8 ± 1.2 °C vs. 37.07 ± 1.1 °C) 4 h after the administration of protamine (T5) (P=0.09), nearly, normalized in T7 and T8. The relation PaO
2/FiO
2was similar, in both groups. The drop in T7 compared to T5 (246.38 ± 129.39 vs. 224.14 ± 165.71) was significant only in the Control Group (
P = 0.04). Bleeding, in the first 48h of PO, was similar in both groups (17.6 vs. 18.1 ml/kg) (
P > 0.05). One patient of the Aprotinin Group was transfused with concentration of erythrocytes (10 ml/kg), in T5, for anemia, according protocol of PICU. There was higher infusion of human albumin in the Aprotinin Group (27.58 ± 30.27 vs. 12.95 ± 18.58 ml/kg), however, not statistically significant (
P>0.05). The concentration of platelets (12 ml/kg) was used in two patients in the Control Group (T6 and T7, respectively). The number of hemoderivative donors to which the children of both groups were exposed was similar (median of 2).
The diuresis, up to T5 (4 h after protamine), was similar in both groups (197 ± 78 vs. 221 ± 223 ml/kg;
P = 0.39), followed by discrete drop in T6 (11.2 ± 5.6 vs. 14.7 ± 5.6 ml/kg; P = 0.16), with significant subsequent increase, in both groups, in T8 (43.0 ± 13.8 vs. 69.7 ± 32.4;
P = 0.19) (Figure 2). In T5, there was large similarity between groups regarding uremia (18.0 ± 5.2 vs.17.4 ± 6.9 g/dl) and plasmatic concentration of creatinine (0.3 ± 0.1 vs. 0.3 ± 0.1 mg/dl), that increased significantly from T5 to T8, when uremia corresponded to 37.2 ± 11.5 vs. 28.0 ± 11.4 g/dl (
P<0.01 for both groups), and the plasmatic concentration of creatinine, at 0.56 ± 0.15 vs. 0.48 ± 0.22 mg/dl (
P<0.01 and P=0.02, respectively). There was no difference between groups (
P>0.05). The depuration of creatinine remained stable in the first 24h of postoperative (
P=0.36). On the second day of PO, it was observed drop below 50 ml/min/1.73 m² in 3 patients of the Aprotinin Group and in 2, of the Control Group, with positive evolution, with no need for dialysis.
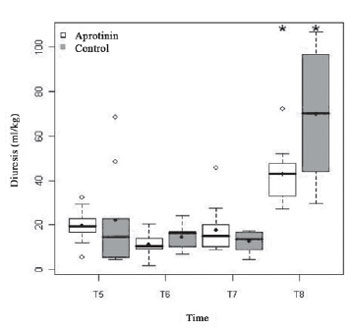
Fig. 2 - Diuresis (ml/kg) of the Aprotinin and Control Groups, in times T5 a T8. The statistically significative intergroup differences are displayed
The rates of hemoglobin (9.6 ± 1.1 vs. 8.9 ± 1.3; P=0.40) and basal hematocryt (29.3 ± 2.8 vs. 27.5 ± 4.2%;
P=0.49) were lower in T1, in the Control Group, with no significant difference. Both groups had significant drop from T1 to T2 (7.6 ± 1.9 vs. 9.59 ± 1.5 g/dl and 23.4 ± 5.6 vs. 29.5 ± 3.9%), followed by progressive hemoconcentration up to T5 (12.1 ± 1.9 vs. 12.7 ± 2.9 g/dl and 37.6 ± 4.9 vs. 40.2 ± 8.6%), which value was statistically higher than T1 (
P<0.01). In T2 and T3, the hemoglobin and hematocryt were significantly lower in the Aprotinin Group (
P<0.01 and P<0.05, respectively) (Figure 3).
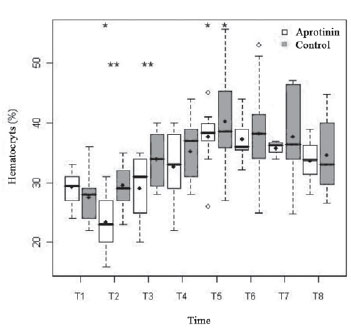
Fig. 3 - Hematocryt rates (%) in the Aprotinin and Control Groups, in times T1 a T8. The statistically significative differences are displayed
Regarding the myocardial markers, the basal serum levels of cTnI (Figure 4) were similar in both groups (
P=0.98) and increased, noticeably, with the ECC, in both groups with peaks from T4 to T6 (
P<0.01) (Table 3). There was no difference between groups from T2 to T8 (
P>0.12). The serum concentration of CK-MB was similar in both groups, 4 h (100.01 ± 51.10 vs. 94.34 ± 44.72 U/l) and 12 h (69.97 ± 14.94 U/l vs. 62.51 ± 30.93) after administration of protamine (
P>0.36).
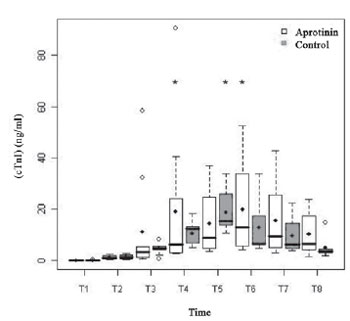
Fig. 4 - Cardiac troponine I (cTnI) (ng/ml), in the Aprotinin and Control Groups. The statistically significative intragroup differences are displayed
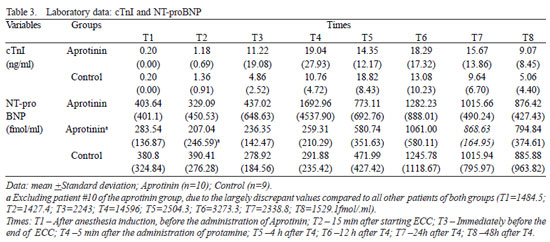
The basal serum levels of Nt-proBNP (fmol/ml) (Figure 5) showed supranormal and similar in both groups (P=0.66), with 45% of the children presenting values higher than 400 fmol/ml (Table 2). Both groups had behavior nearly similar, with progressive increase from T4 to T6, when they reached statistical significance (
P<0.01). There was difference between groups in T2 (
P=0.04), after excluding the statistical analysis one patient of the Aprotinin Group, with values largely discrepant. The dosage of glutamic oxalacetic transaminase enzyme (GOT) was similar in both groups, 4 (106,18 ± 41,96 vs. 92,37 ± 44,72) e 12 h (107,00 ± 54,78 vs. 98,42 ± 37,49) after administration of protamine (
P>0.30).
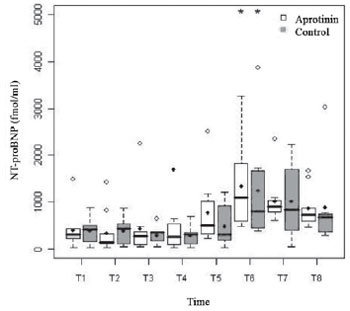
Fig. 5 - Levels of NT-proBNP (fmol/ml) in the Aprotinin and Control Groups, in times T1 to T8. The statistically significative intragroup differences are displayed
The basal levels of arterial serum lactate were similar in both groups (0.98 ± 0.24 vs. 1.35 ± 0.51 mmol/l;
P=0.09) and were both followed by accentuated increase, with the start of ECC (T2) (3.67 ± 2.00 vs. 3.11 ± 2.83 mmol/l;
P<0.01). In both groups, there was a second peak, 5 min after administration of protamine (T4) (3.67 ± 2.00 vs. 2.89 ± 1.45;
P<0.01). In the postoperative, the lactatemia decreased in both groups until normalizing in 48 hours (Figure 6). The hyperlactatemia was statistically significant from T2 to T8 in the Aprotinin Group (
P<0.01), and from T2 to T5 in the Control Group (
P<0.01). There was statistically significant difference between groups in the times T3, T4 and T6.
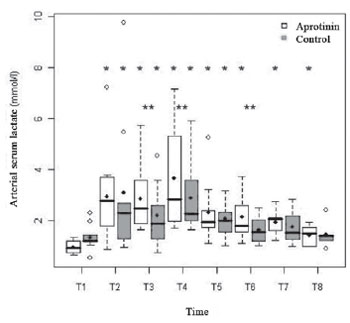
Fig. 6 - Lactatemia (mmol/l) in the Aprotinin and Control Groups, in times T1 a T8. The statistically significative intragroup and intergroup differences are displayed
After anesthetic induction (T1), the arterial blood pH was significantly lower (7.31 vs. 7.43;
P=0.01) and the PaCO
2 was higher and supranormal in the Aprotinin Group (48.03 ± 15.95 vs. 38.12 ± 5.45 mmHg), although with no significant difference between groups (
P=0.14).
In the Aprotinin Group, 50% of the patients presented respiratory acidosis in T1 (pH = 7.23 ± 0.10 and pCO2 = 58.7 ± 16 mmHg), which did not occur in the Control Group. The pH showed differences between groups in T4 (P=0.04), and the PaCO
2 presented significant drop in the Aprotinin Group, 4 and 12 h after protamine administration (T5 and T6) (33.48 ± 9.17 and 34.60 ± 7.18 mmHg, respectively) compared to T1 (
P<0.01 and
P=0.02, respectively). The difference between groups of PaCO
2, in T5, was statistically significant (
P=0.02), with respiratory alkalosis in six patients of the Aprotinin Group and respiratory acidosis in two patients of the Control Group. In T5 and T6, 70% of the patients of the Aprotinin Group, and 66.6% and 55.5%, respectively, of the patients of the Control Group, were submitted to mechanical pulmonary ventilation. The PaCO
2 was normal in both groups in 24 and 48 hours of PO.
In the Aprotinin Group, there was significant drop of the arterial concentration of arterial plasmatic bicarbonate, from T5 to T7, with nadir in T6 (18.94 ± 2.02 mmol/l;
P<0.01), whereas, in the Control Group, the nadir also occurred significantly in T6 (21.42 ± 3.22 mmol/l;
P=0.02). Metabolic acidosis occurred in T6 in both groups, especially in the Aprotinin Group (6 vs. 2 patients). Nevertheless, there was no statistical difference between groups (
P=0.69) (Figure 7).
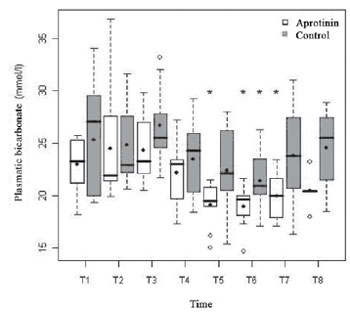
Fig. 7 - Arterial Concentration of bicarbonate (mmol/l) in the Aprotinin and Control Groups, in times T1 a T8. The statistically significative intragroup differences are displayed
In both groups, occurred cardiovascular dysfunctions (60 vs. 33.3%), renal dysfunctions (30 vs. 22.2%) and respiratory dysfunctions (40 vs. 22.2%), with no statistically significant difference (
P>0.05). Two children in the Control Group presented hematologic dysfunction. There were no cases of neurolgical or hepatic dysfunction. Infectious pulmonary complications occurred in both groups (20 vs. 33.3%).
The times of hospital and PICU internment were not altered significantly by organic dysfunctions observed. There were no deaths. All patients were released from hospital, in fair clinical and cicatrisation conditions. The postoperative Doppler echocardiographic evaluation showed positive surgical results in all patients. There were no such problems as allergy, low blood pressure or thrombosis as adverse effects of aprotinin.
DISCUSSION
In this study, our purpose was to evaluate the impact and the consequences of using aprotinin in high doses over clinical evolution and intraoperative and postoperative metabolic variables, as well as over myocardial and renal serum markers, in acyanotic children submitted to surgical procedures of same complexity and similar times of ECC and anoxia. The use of aprotinin in cardiac surgery was a controversial issue regarding costs and benefits, for it was held to be unfeasible due to its high cost and adverse effects in low risk cases, thus, not being considered the drug of first choice. However, it was referred as an antifibrinolytic of evidence class A level 1 with proved efficiency and potential utility for its use in children predisposed to capillary hyperpermeability syndrome, excessive bleeding and postoperative myocardial failure [1-4,8], as the ones operated in this study, of low weight, generally due to a serious degree of malnutrition. These considerations raised the present investigation, once that the aprotinin was largely used in our cases observing the recent reports of its association with high cardiac, cerebral and renal risks, as well as mortality in adults.
The age range was restricted between 30 days and four years, aiming to reduce age dispersion, which blinds similar studies. Neonates were excluded, due to higher possibility of ECC complications, secondary to the intense neuro-endocrinal response to tissue lesion [1,18].
The presence of respiratory acidosis after the anesthetic induction, in patients of the aprotinin group evidences the existence of serious cases of ICC, with intense hyperflow and pulmonary congestion, determinants of probable interstitial edema in the organ, with higher risk of death [20,21]. Nonetheless, the mortality estimative inside the hospital or 30 days after release, from 1 to 5%, based on the risk scores more evident (RACHS-1 and Aristotle), no deaths occurred in this study. This positive surgical result was enforced by the most recent clinical-surgical index proposed in Brazil [18], which scores (5.5 vs. 5) correspond to risks of death from 11.70% to 23.98%, , mainly, due to the prevalence of low weight, indicative of malnutrition, and genetic syndromes, pulmonary high blood pressure and ICC, that certainly influenced morbidity.
As a belief that aprotinin reduces bleeding and the need of transfusions by a dose-dependent device [2], we decided on the use of the first protocol (Hammersmith Hospital), denominated high dose, proportional to the body surface, aiming to enhance the clinical benefits. Unfortunately, the dosis, certainly, had the side effect of introducing a flank of higher hemodilution in the group treated, as observed by the accentuated drop in the levels of hemoglobin and albuminemia, once the volumes of volumes of priming, hemofiltrated and cardioplegia, as well as the concentration of erythrocytes, diuresis and times of perfusion and anoxia being similar to those of the Control Group. There was similarity in total postoperative bleeding between the groups, below the reported in the literature [2], however, the hematological dysfunction (platelet depletion with bleeding) occurred only in the Control Group (2 cases).
As expected, the preoperative serum concentrations of troponine-I and lactate were normal, indicating that no patient presented myocardial ischemia or terminal cardiac insufficiency. The PRISM score, upon entering the PICU (3 vs. 7) suggested that the drug promoted metabolic and hemodynamic initial stability in the Aprotinin Group, but the clinical evolution of the groups did not differ. The similarity of the groups regarding the duration, associations and dose of inotropics in the first 48h after ECC, a well as the times of mechanical pulmonary ventilation and stay in PICU, implicates that the aprotinin did not influence favorably in these clinical variables. This does not relate to the studies in which the aprotinin reduced the use of inotropics [7], the incidence of low debit syndrome and arrhythmias [8], stay in PICU, hospital internment and duration of mechanical ventilation [2,4]. In the Aprotinin Group, however, there was a tendency to higher incidence of organic dysfunctions, either cardiovascular (60% vs. 33.3%) or respiratory (40% vs. 22.2%), although with no statistically significant difference. These rates, however, are expected in the age group, for it is acceptable that 50% of the patients present pulmonary and cardiovascular dysfunctions [22].
The inotropic score and the volemic reposition tended to increase in the aprotinin group, perhaps, as undesirable side effects of higher hemodilution to which it was submitted. Furthermore, this tendency to higher inotropic scores in children operated with ECC using aprotinin was reported recently, with higher scores in simpler heart diseases, being paradoxically lower the need of inotropic support in more complex heart diseases [23].
Taking into account that the cardiac incisions and the times of ECC and aortic clamping were similar, there were no significant differences between groups in the values of cardiac troponin I (cTnI). On the other hand, cTnI, the absence of myocardial-protecting effect of the Aprotinin opposed either the previous reports, indicatives of benefits [7,8] as well as other, in which it was detrimental [9]. The same applies to the dosages of CKMB that did not differ from those of the Control Group, with similar serum profile to those of other studies involving children [24].
The hyperlactatemia is frequently observed in children submitted to cardiac surgery, being that lactic acidosis may occur in 10% of cases [25], generally, due to the intense hemodilution, associated to high blood pressure, in the initial stages of ECC [16]. In this work, the hyperlactatemia, although, generally, considered discrete, from 2 to 4 mmol/l [26], was higher in the Aprotinin Group. According to observations of Koliski et al. [27], in 2005, the highest level of lactate, at the end of ECC and 5 min after administration of protamine, it may have occurred from hypoperfusion and inadequate tissue oxygenation following intraoperative hemodilution, that probably was not sufficiently eliminated by the arterial flow and by the reposition of the concentration of erythrocytes during ECC, with hemoconcentration close to the end of ECC. Usually, the levels of lactate are lower when using blood primming [16,28]. The values were similar to those of Carmona [24], in 2006, especially in children without myocardial dysfunction. It may have occurred influence of Aprotininin this finding, although this possibility has not been detected by Wippermann et al. [7], in 1999. The hyperlactatemia 12 hours after protamine administration, in the Aprotinin Group, with normalized hemoglobin, may have resulted from better tissue perfusion, triggered by increase in anaerobic glycolisis induced either by catecholamines [26] or by a paradoxal effect originated from the tissue wash out resulting from the postoperative volemic re-expansion and peripheral vasodilation [25].
Hatherill et al. [29], in 1997, stressed that several factors of confusion interfere in lactatemia, with the reduction in tissue oxygen extraction, the unbalance between production and hepatic clearance, and the technique and duration of perfusion and anesthesia. There was no hepatic dysfunction clinically evident, although, in the laboratory, there was an increase in GOT, but it did not differ between the groups. The duration of anesthesia and perfusion was similar between the groups. The metabolic acidosis observed in the period from 4 to 12 hours after surgery, especially in 6 out of 10 patients of the Aprotinin Group, may not be due to the lactate levels (< 4 mmol/l). A possible explanation consists in eventual hypercloremic acidosis or increase of tissue acids not measured [25], observing that hipoalbuminemia, presenting our children, may underestimate the interpretation of the degree of metabolic acidosis [30]. Based on experimental findings of Marin-Grez et al. [31], in1995, it is still possible that the metabolic acidosis may be related to the bloching of renal calicreine by aprotinin. This additional para-effect of the drug requires, however, further investigation.
The NT-proBNP, sensitive neuro-humoral marker of ventricular dysfunction [16], comes from the ventricles, in the shape of an inactive pro-hormone (pro-BNP). From the enzymatic cleavage of the latter, in the blood current, result the BNP biologically active and the marker, as inactive residue. The levels of NT-proBNP increase in congestive cardiac insufficiency and in congenital heart diseases [16], being possible the up-regulation of the cardiac BNP by inflammatory cytokines [24]. In this research, the detection of high basal rates of NT-proBNP in, basically, half of the cases, certainly, was due to the presence of ICC. The Aprotinin did not interfere in the flowing concentration of this biochemical residue, which concentration increased, similarly, at the end of ECC, with peak 12h after the infusion of protamine, according values acquired by other authors and not suggesting myocardial dysfunction [16,24].
The significant decrease in the postoperative relation PaO
2/FiO
2, in the Control Group, compatible with acute pulmonary lesion, was not significant in the Aprotinin Group, inferring protective effect of the Aprotinin, although clinically inefficient, for there was no significant difference in the time of mechanical ventilation. This observation is contrary to the report of Mössinger et al. [2], in 2003 that observed reduction in the time of mechanical ventilation using the drug.
There were no significant differences between the groups regarding diuresis, serum levels of urea and creatinine, depuration of creatinine and occurrence of acute renal insufficiency. These findings, although superposed to several studies in children [2,24,32] and adults, contrast with those that detected injure in the renal function [9]. In recent retrospective studies of children operated with ECC, there was no association between the use of aprotinine and IRA, dialysis, neurological complications and mortality [12], being reported that the time of ECC over 100 minutes was the main marker of the postoperative renal dysfunction in neonates and not the use of drugs [13]. We stress that the time of ECC was less than 100 minutes in our patients. In another study, performed by Székely et al. [23], in 2008, although a higher rate of renal dysfunction and dialysis in a group treated with aprotinin than on the total group, in 657 children under 18 years-old, there was no significant association and regardless the drug after adjustment of the risk rates in the clinical variables and procedures performed, which was also reported by Manrique et al. [14], in 2009, in 395 children (being 67 neonates). In another recent and prospective study in neonates there were also no renal complications found [15]. It was also reported that the levels of urinary aprotinin 2 hours after the start of ECC were excellent early biomarkers for the subsequent development acute renal lesion and its complications, being, also, its monitoring a possible prophylactic strategy of diagnostic for postoperative renal dysfunction [33].
Though, the limitation of our study being the small series of cases for comparison between the groups, becoming necessary to increase the number of samples, however, nowadays it is difficult due to the unavailability of the aprotinin in the market. On the other hand, it was used the most powerful test for sample compared to the non-parametric test, being that the statistical analysis through the referred model (multiple regression), with fix effects (fix group and time) and random (particularities of each child), allowed to show that the sample was feasible for the analysis. The risk is not detecting a possible significance between the groups that may exist in case the sample was larger, as in bleeding. The error that might occur is the type I. The sureness is 95%. Hence, the size of the sample may justify some of the adverse results. There were found in this study evidences of adverse effects related to higher hemodilution, with a tendency to higher levels of serum lactate and metabolic acidosis and with evidences, statistically not significant, of cardiovascular and respiratory dysfunction and inotropic scores more accentuated in the Aprotinin Group compared to the Control Group. Amplifying the sample is, therefore, necessary, to attain a better explanation of these effects, in multicenter studies and/or in surgical groups with a larger number children operated for this type of heart disease, although difficult nowadays due the unavailability of the aprotinin in the market.
CONCLUSIONS
Aprotinin in high haemostatic doses did not influence significantly the serum markers s troponine I, NT-proBNP and of renal function, however, it was associated to higher hemodilution, lactatemia and metabolic acidosis.
1. Wegner J. Biochemistry of serine protease inhibitors and their mechanisms of action: a review. J Extra Corpor Technol. 2003; 35(4): 326-38. [MedLine]
2. Mössinger H, DietrichW, Braun SL, Jochum M, Meisner H, Richter JA. High-dose aprotinin reduces activation of hemostasis, allogeneic blood requirement, and duration of postoperative ventilation in pediatric cardiac surgery. Ann Thorac Surg. 2003;75(2):430-7. [MedLine]
3. Arnold DM, Fergusson DA, Chan AK, Cook RJ, Fraser GA, Lim W, et al. Avoiding transfusions in children undergoing cardiac surgery: a meta-analysis of randomized trilas os aprotinin. Anesth Analg. 2006;102(3):731-7. [MedLine]
4. Twite MD, Hammer GB. The use of aprotinin in pediatric cardiac surgery: should we bid 'good riddance' or are we throwing out the baby with the bath water? Paediatr Anaesth. 2008;18(9):809-11. [MedLine]
5. Clasen C, Jochum M, Mueller-Esterl W. Feasibility study of very high aprotinin dosage in polytrauma patients. Prog Clin Biol Res. 1987;236A:175-83. [MedLine]
6. Khan TA, Bianchi C, Voisine P, Feng J, Baker J, Hart M, et al. Reduction of myocardial reperfusion injury by aprotinin after regional ischemia and cardioplegic arrest. J Thorac Cardiovasc Surg. 2004;128(4):602-8. [MedLine]
7. Wippermann CF, Schmid FX, Eberle B, Huth RG, Kampmann C, Schranz D, et al. Reduced inotropic support after aprotinin therapy during pediatric cardiac operations. Ann Thorac Surg. 1999;67(1):173-6. [MedLine]
8. Broche F, Romero A, Olembe E, Céspedes E, Peña M, Romay C, et al. Aprotinin mediated antioxidant effect in Cardiosurgery with mechanical cardiorespiratory support (CMCS). J Cardiovasc Surg (Torino). 2002;43(4):429-36. [MedLine]
9. Mangano DT, Tudor IC, Dietzel C; Multicenter Study of Perioperative Ischemia Research Group; Ischemia Research and Education Foundation. The risk associated with aprotinin in cardiac surgery. N Engl J Med. 2006;354(4):353-65. [MedLine]
10. Fergusson DA, Hébert PC, Mazer CD, Fremes S, MacAdams C, Murkin JM, et al. A comparison of aprotinin and lysine analogues in high-risk cardiac surgery. N Engl J Med. 2008;358(22):2319-31. [MedLine]
11. Checchia PA, Bronicki RA, Costello JM, Nelson DP. Steroid use before pediatric cardiac operations using cardiopulmonary bypass: an international survey of 36 centers. Pediatr Crit Care Med. 2005;6(4):441-4. [MedLine]
12. Backer CL, Kelle AM, Stewart RD, Suresh SC, Ali FN, Cohn RA, et al. Aprotinin is safe in pediatric patients undergoing cardiac surgery. J Thorac Cardiovasc Surg. 2007;134(6):1421-6.
13. Guzzetta NA, Evans FM, Rosenberg ES, Fazlollah TM, Baker MJ, Wilson EC, et al. The impact of aprotinin on postoperative renal dysfunction in neonates undergoing cardiopulmonary bypass: a retrospective analysis. Anesth Analg. 2009;108(2):448-55. [MedLine]
14. Manrique A, Jooste EH, Kuch BA, Litchtenstein SE, Morell V, Munoz R, et al. The association of renal dysfunction and the use of aprotinin in patients undergoing congenital cardiac surgery requiring cardiopulmonary bypass. Anesth Analg. 2009;109(1):45-52. [MedLine]
15. Williams GD, Ramamoorthy C, Pentcheva K, Boltz MG, Kamra K, Reddy VM. A randomized, controlled trial of aprotinin in neonates undergoing open-heart surgery. Paediatr Anaesth. 2008;18(9):812-9. [MedLine]
16. Gessler P, Knirsch W, Schmitt B, Rousson V, von Eckardstein A. Prognostic value of plasma N-terminal pro-brain natriuretic peptide in children with congenital heart defects and open-heart surgery. J Pediatr. 2006;148(3):372-6. [MedLine]
17. Saraiya NR, Sun LS, Jonassen AE, Pesce MA, Queagebeur JM. Serum cardiac troponin-I elevation in neonatal cardiac surgery is lesion-dependent. J Cardiothorac Vasc Anesth. 2005;19(5):620-5. [MedLine]
18. Mattos SS, Neves JR, Costa MC, Hatem TP, Luna CF. An index for evaluating in paediatric cardiac intensive care. Cardiol Young. 2006;16(4):369-77. [MedLine]
19. Goldstein B, Giroir B, Randolph A; International Consensus Conference on Pediatric Sepsis. International pediatric sepsis consensus conference: definitions for sepsis and organ dysfunction in pediatrics. Pediatr Crit Care. Med. 2005;6(1):2-8. [MedLine]
20. Abellan DM, Gimenez SC. Insuficiência cardíaca congestiva: diagnóstico e tratamento. In: Santana MVT, ed. Cardiopatias congênitas no recém-nascido: diagnóstico e tratamento. 1ª ed. São Paulo:Atheneu;2000. p.102-14.
21. Moura MR. Emergências em cardiologia pediátrica. In: Macruz R, Snitcowsky R, eds. Cardiologia pediátrica. 1ª ed. São Paulo:Sarvier;1988. p.657-74.
22. Hoffman TM, Wernovsky G, Atz AM, Kulik TJ, Nelson DP, Chang AC, et al. Efficacy and safety of milrinone in preventing low cardiac output syndrome in infants and children after corrective surgery for congenital heart disease. Circulation. 2003;107(7):996-1002. [MedLine]
23. Székely A, Sápi E, Breuer T, Kertai MD, Bodor G, Vargha P, et al. Aprotinin and renal dysfunction after pediatric cardiac surgery. Paediatr Anaesth. 2008;18(2):151-9. [MedLine]
24. Carmona F. Avaliação dos fatores de risco para disfunção miocárdica e mortalidade intra-hospitalar em neonatos e lactentes submetidos a cirurgia cardíaca com circulação extracorpórea [Dissertação de Mestrado]. Ribeirão Preto: Faculdade de Medicina de Ribeirão Preto da Universidade de São Paulo;2006. 153p.
25. Murray DM, Olhsson V, Fraser JI. Defining acidosis in postoperative cardiac patients using Stewart's method of strong ion difference. Pediatr Crit Care Med. 2004;5(3):240-5. [MedLine]
26. Atik FA. Monitorização hemodinâmica em cirurgia cardíaca pediátrica. Arq Bras Cardiol. 2004;82(2):199-208. [MedLine]
27. Koliski A, Cat I, Giraldi DJ, Cat ML. Lactato sérico como marcador prognóstico em crianças gravemente doentes. J Pediatr (Rio J). 2005;81(4):287-92. [MedLine]
28. Toda Y, Duke T, Shekerdemian LS. Influences on lactate levels in children early after cardiac surgery: prime solution and age. Crit Care Resusc. 2005;7(2):87-91. [MedLine]
29. Hatherill M, Sajjanhar T,Tibby SM, Champion MP,Anderson D, Marsh MJ, et al. Serum lactate as a predictor of mortality after paediatric cardiac surgery. Arch Dis Child. 1997;77(3):235-8. [MedLine]
30. Hatherill M, Waggie Z, Purves L, Reynolds L, Argent A. Correction of the anion gap for albumin in order to detect occult tissue anions in shock. Arch Dis Child. 2002;87(6):526-9. [MedLine]
31. Marin-Grez M, Vallés P, Odigie PI. Involvement of renal kallikrein in the regulation of bicarbonate excretion in rats. J Physiol. 1995;488(Pt 1):163-70. [MedLine]
32. Skidmore KL, Azakie A. Aprotinin and sevoflurane do not affect renal function during single-ventricle palliative surgery. Anesth Analg. 2006;103(6):1614-5. [MedLine]
33. Nguyen MT, Dent CL, Ross GF, Harris N, Manning PB, Mitsnefes MM, et al. Urinary aprotinin as a predictor of acute kidney injury after cardiac surgery in children receiving aprotinin therapy. Pediatr Nephrol. 2008;23(8):1317-26. [MedLine]